Introduction
Electrical energy storage (EES) systems constitute an essential element in the development of sustainable energy technologies. Electrical energy generated from renewable resources such as solar radiation or wind provides great potential to meet our energy needs in a sustainable manner. However, these renewable energy technologies generate electricity intermittently and thus require efficient and reliable electrical energy storage methods. For commercial and residential end-use, electricity must be reliably available at any time of the day. In fact, second-to-second
fluctuations can cause major disruptions with costs estimated to be in the tens of billions of dollars annually. Thus, the development of new EES systems will be critical in the use of large-scale solar
or wind-based electricity generation. Moreover, greatly improved EES systems are required to enable the widespread use of hybrid electrical vehicles (HEV),
plug-in hybrids, and all-electric vehicles. Improvements in ESS performance, reliability, and efficiency are needed in the development of modern portable electronic devices such as laptops and smart phones.
Electrochemical Capacitors
Electrochemical capacitors (ECs), also known as supercapacitors or ultracapacitors, are typically classified into two categories based on their different energy
storage mechanisms, i.e., electric double layer capacitors (EDLCs) and pseudocapacitors. First, EDLCs store charges physically in electric double layers forming near the electrode/electrolyte interfaces. Thus, the process is highly reversible and the cycle life is essentially infinite. On the other hand, pseudocapacitors store energy via not only electric double layer such as that in EDLCs but also fast surface oxidation-reduction (redox) reactions as well as possible ion intercalation in the electrode.
The performance of electrochemical capacitors is determined by the combination of the electrode material and electrolyte used. There are three main categories of electrode materials used for ECs, namely (1) carbon-based materials, (2) transition metal oxides, and (3) conductive polymers. Similarly, three types of electrolyte materials are used for ECs including (1) aqueous electrolytes, (2) organic electrolytes, and (3) ionic liquids. |
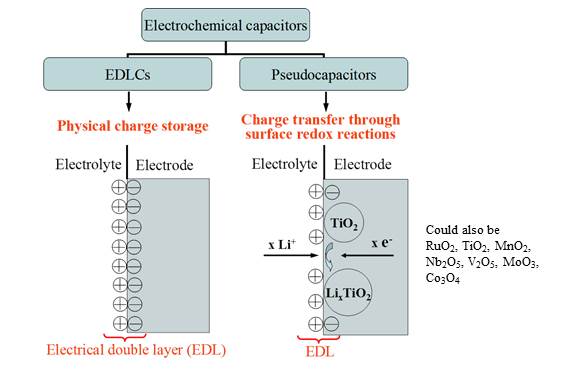
Fig. 1. Classification of electrochemical capacitors into electrical double layer capacitors and pseudocapacitors. |
Ragone plot
The performances of ECs can be compared in the Ragone chart plotting their respective energy and power densities as illustrated in Fig.2 for different electrical energy storage devices. Due to their physical charge storage, capacitors feature very large power densities compared with batteries and fuel cells but low energy densities. On the other hand, batteries and fuel cells have large energy densities but low power densities due to their slow reaction kinetics. Electrochemical capacitors bridge the gap between capacitors and batteries/fuel cells. They offer the prospect of maintaining the high energy density of batteries without compromising the high power density of capacitors. |
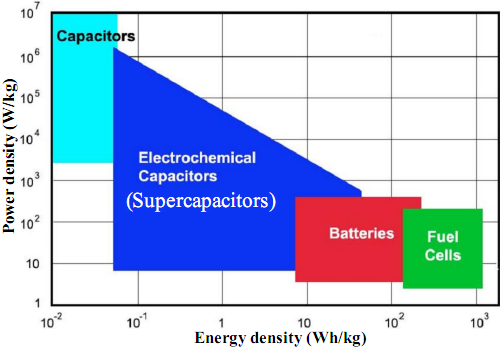
Fig.2. Power density vs. energy density of various energy storage systems. |
|
Objectives
Understanding the combined effects of interfacial, transport, and electrochemical phenomena taking place in electrochemical capacitors is essential to their development.Our laboratory effort aims (1) to develop rigorous
and experimentally validated physical modeling of electrochemical capacitors, (2) to identify the dominant physical phenomena controlling the behavior of electrochemical capacitors, and (3) to formulate design rules for the porous electrode morphology and for the electrolyte.
|